Soft materials are highly correlated many body systems with complex structures and dynamics spanning a wide range of length and time scales. Their relaxation processes
involve complex phenomena such as vitrification, jamming, entanglement and semi-crystallization, which are highly process-dependent and for which no comprehensive theoretical
framework and understanding exist. Optimal use of soft materials in future electronics, medicine and energy devices demand a deeper understand of these phenomena. Our
objectives are to improve the fundamental understanding of these phenomena in polymer and other soft matter systems, and establish their design rules for engineering applications.
A central barrier in improving the fundamental understanding and design of soft materials is the unavailability of transferable potential models that accurately describe
inter-particle interactions in soft matter systems for a wide range of physicochemical conditions. The current fixed functional form based approaches pose limitation on the
physics and chemistry that can be captured. On the other hand, fully atomistic models are limited by their short time and length scales. To address these limitations, we
develop fully flexible coarse-grained deep neural network potentials that capture polymer dynamics accurately, and conduct large-scale molecular simulations for predicting
macroscopic properties.
Polymeric ionic liquids (PILs) are of considerable interest as next-generation battery materials due to their potential to combine the solid-like stability of polymers with
the high ion conductivity of ionic liquids (ILs). However, polymerization of ionic liquids to form a polymer generally leads to a suppression in ion transport rate that has
proven to be a major barrier to the realization of commercially viable PIL solid electrolytes. Hence, the development of environmentally stable high density energy storage
materials demands addressing few fundamental polymer physics problems. First, the conductivity of ions is appeared to be intimately coupled with the segmental dynamics of polymers.
Second, extensive evidences suggest an inverse relationship between conductivity and the modulus of PILs. Third, both ion conductivity and mechanical properties of ILs and PILs are
strongly related to their glass forming behavior. These factors represent a major challenge to the rational design of polymer electrolytes as the next generation energy storage
materials. Our aim is to combine computer simulations and machine learning to develop deeper understanding of these correlations and identify strategies by which ion conductivity
can be maximized by maximizing both PIL segmental relaxation rates and the extent of ion transport decoupling from chain dynamics.
Advances in scalable self-assembly strategies have enabled synthesis of highly ordered arrays of polymer grafted colloidal nanoparticles (NPs), termed nanoparticle superlattices
(NPSLs), with exceptional thermal, mechanical, electronic, and optical properties. For instance, precise ordering of NPs into NPSLs has led to several technologically relevant phenomena
including reversible metal-to-insulator transition, vibrational coherence, enhanced p-type conductivity, ferro-/ferri-magnetism, novel plasmonic properties, spin-dependent electron
transport, and superior thermal behavior. It has long been suspected that the structure and dynamics of polymers detect the long-range order and properties of NPSLs. But, the correlations
between polymer dynamics and thermophysical properties of NPSLs are not fully understood. We employ molecular simulations of generic polymer and nanoparticle models to develop a
comprehensive understanding of the interrelationship between polymer dynamics and thermophysical properties of NPSLs. Our work focuses on understanding the role played by polymer
dynamics in dictating the symmetry of special ordering of nanoparticles in a NPSL, and the influence of polymer density/distribution on the properties of superlattice. We study a
wide range of nanoparticles – cube, sphere, rod, tetrahedron and plates in order to develop universal correlations between structural order of NPSLs and polymer dynamics.
Deep Neural Netork Models for Soft Materials
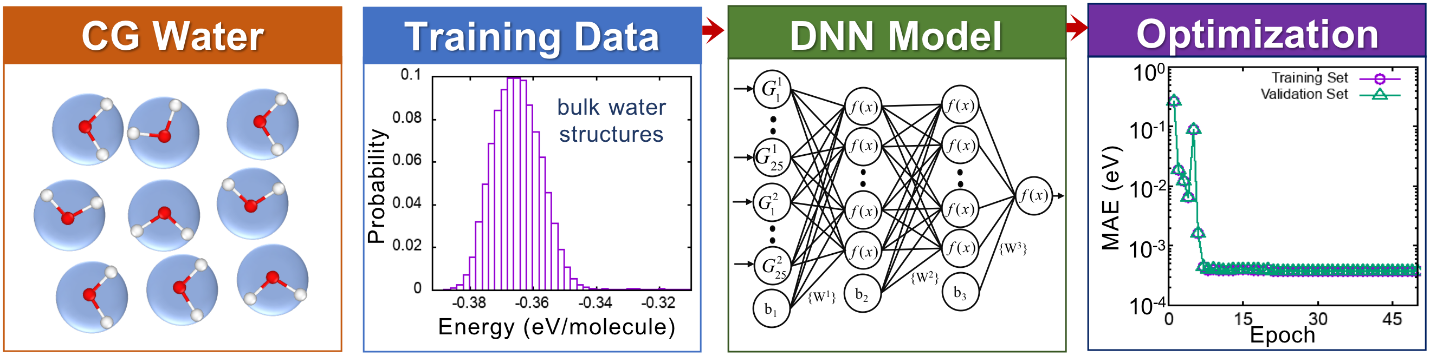
Polymeric Ionic Liquids
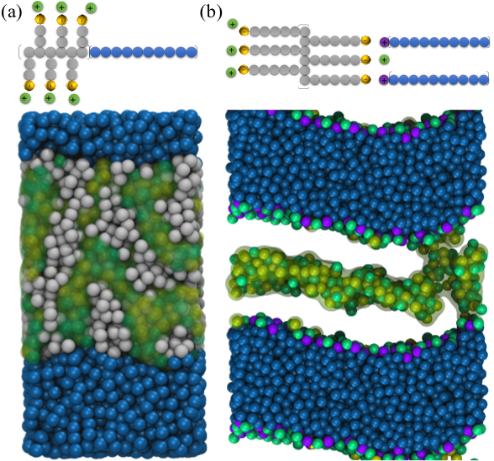
Nanoparticle Superlattics
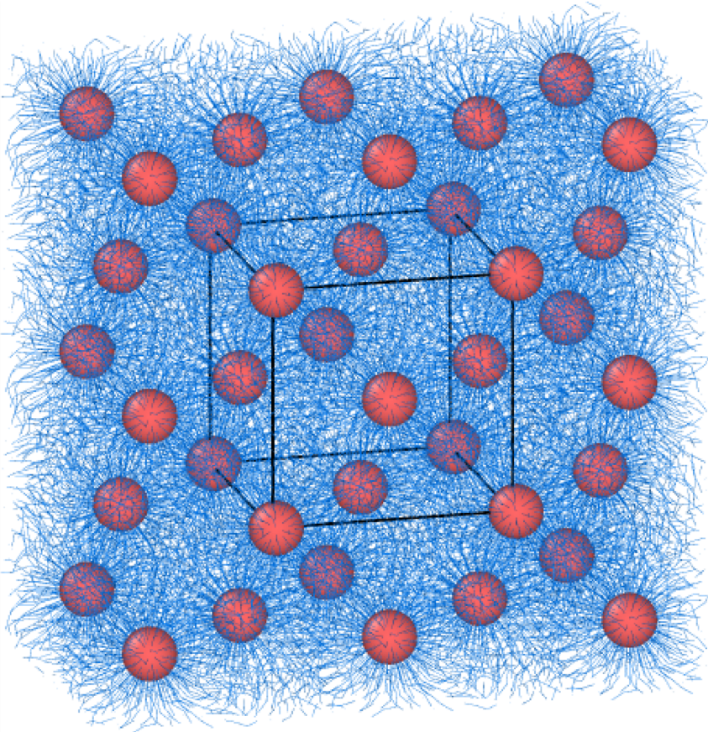
Recent News :
- Three of our group members have received exciting job offers. Details in the News page.
- The group receives one million hours of computing time on Theta of Argonne Leadership Computing Facility. We gratefully acknowledge Argonne National Laboratory for providing us access to its leadership class computing facility.
Reach out
NAC 447, IIT Madras, Chennai, TN 600036 Phone:91 44 2257 4162
E-mail: tpatra@iitm.ac.in